Brake pads are some of the most mysterious and misunderstood car components. On the face of it, all they do is slow your wheels down by converting kinetic energy (motion) to thermal energy (not motion).
But dig deeper and you’ll realize that they have a ton of science and engineering working behind the scenes. In fact, they’re just as chemically complex as tires and catalytic converters.
In this article, we’ll give you the low-down on brake pads by discussing everything from friction materials, compounds, features and quality assessment info that’ll help you make an informed purchase decision.
Understanding Brake Pads: A Precursor
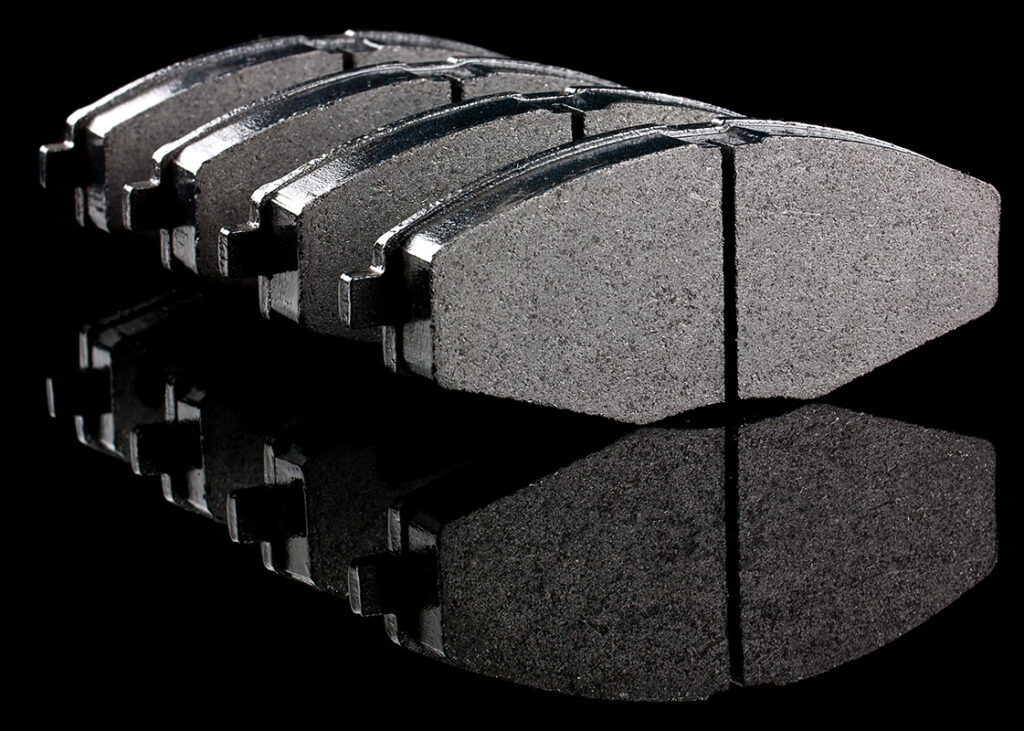
Torque, horsepower, and acceleration are all great, but deceleration is just as important. Being able to brake later and harder will literally put you ahead of the curve, and part of what makes that happen is great brake pads.
Though upgrading your brake pads may not be as sexy as installing new coilovers or air suspension, it can significantly improve your laptimes. Even more so if throw aftermarket rotors in the mix.
All that said, choosing the right ones can be confusing, particularly if you enjoy nerding out on the different types of brake pad compounds and their respective attributes.
For instance, some produce more dust, some perform better at higher temperatures, and some eat through rotors, while others are more sacrificial as they wear out faster in favor of performance.
What they all have in common is that they’re supposed to overcome the extreme torque and heat produced by spinning rotors. How they do it is where the cocktail of friction materials and compounds comes into play.
These friction materials typically adhere to or riveted on to a steel backing plate; the part that slides into your calipers. Their unique formulation impact and impart the following attributes in your brake pads:
- Compressibility
- Longevity
- Rotor wear
- Noise
- Dust generation
- Coefficient of friction
- Fade resistance
- Operating temperature range
Below, we’ll expand on this list and take a closer look at the different friction materials and compounds used by brake pad manufacturers.
Brake Friction Materials 101
As car enthusiasts, we owe a lot to friction materials; their use in braking and transmission systems is part of what creates the driving experience that we all know and love.
Brake pads, clutch plates, automatic transmission bands, parking brake shoes, and synchronizer rings in manual transmissions all rely on friction materials.
Their function is quite straightforward—producing friction between surfaces to control opposing motion or transferring power between components.
With clutches, friction is required between the clutch plate and the flywheel to disconnect the flow of power to the transmission. And in brake systems, it’s the brake pads that utilize friction to slow down the rotors and consequently the tires.
See below for information on assessing the quality of brake pad compounds and to understand how they’re able to do what they do.
Assessing Quality
When choosing brake pad compounds, consider the following factors to assess their quality and impact on your braking performance.
However, it’s worth mentioning that these factors focus on the material composition of brake pads rather than manufacturing standards, testing protocols, or mechanical design.
Formulation
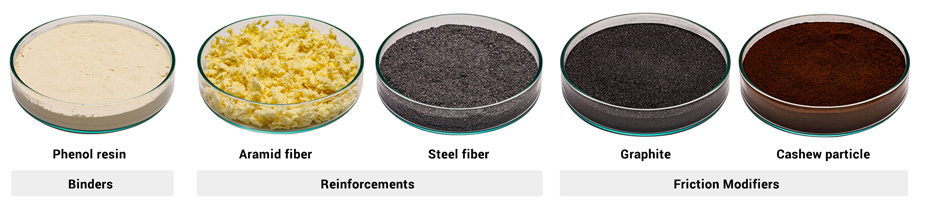
Brake pad or brake lining formulation is both an art and a science. Since well over 20 different kinds of raw materials are involved, blending them and tweaking the proportions to achieve specific results is an unbelievably complex task. It’s like tuning a harpsichord after Mozart has had at it for months, but worse.
Each of the raw materials performs a specific task, like abrasion, lubrication, binding, heat resistance, and providing structural integrity to the amalgam of it all. It’s no wonder that manufacturers protect their brake pad formulations like it’s the recipe for Coca-Cola.
Thermal Conductivity and Fade Resistance
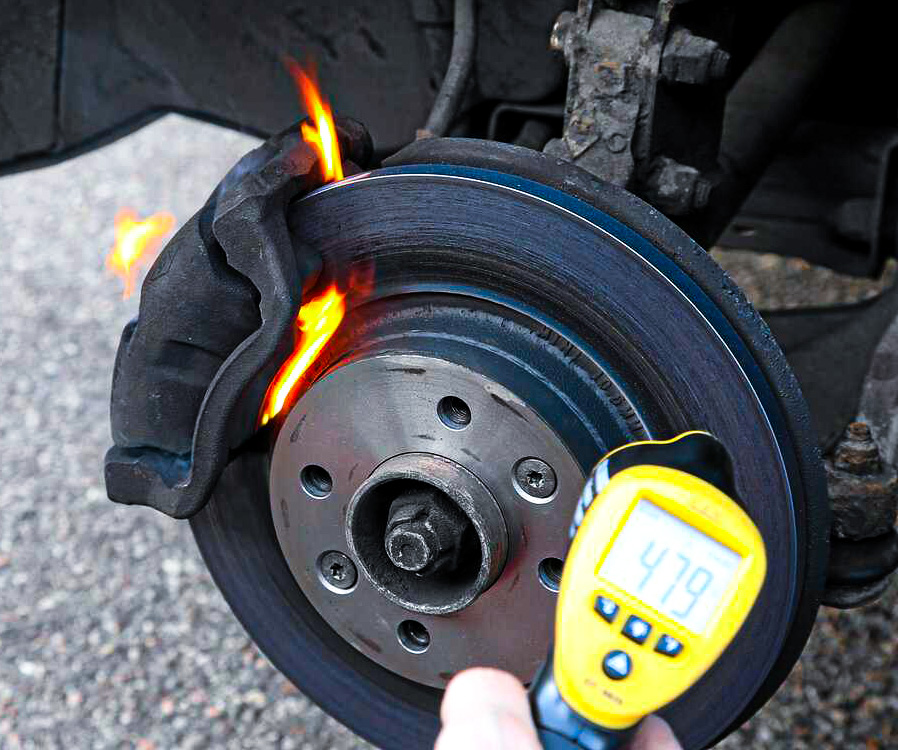
You’ve probably read somewhere that heat is the enemy of performance. While this is true, it only holds ground when talking about extremes. Everything from engines and drivetrain components to brakes and tires has an optimal operating temperature range.
For brake pads to provide any meaningful friction, they require heat. Obviously not to the point where things start melting—balance is key. On the flip side, complaining about “cold brakes” is a thing.
Wondering how friction materials change personalities depending on how much heat is applied to them? Here’s a simple answer: chemistry. When the pads make contact with spinning rotors, the heat generated in the process chemically alters the friction materials and causes them to exhibit peculiar qualities.
As an example, semi-metallic pads perform optimally between 38°C to 371°C, while carbon-carbon composite brakes do bugger all until reaching at least 300°C. These brakes can withstand well over 800°C. It’s only at around 1500°C when thermal degradation becomes a concern.
High-quality brake pads have low thermal conductivity, high resistance to thermal degradation, and consequently fade resistance. The latter refers to when brake pads lose their ability to generate friction when they get too hot.
Materials like semi-metallic, ceramic, and carbon-ceramic pads are far more resistance to brake fade in comparison to organic ones. Most brake pads, regardless of their chemical formulation, feature heat dissipation mechanisms such as slits and cooling channels.
Pad and Rotor Wear Rate
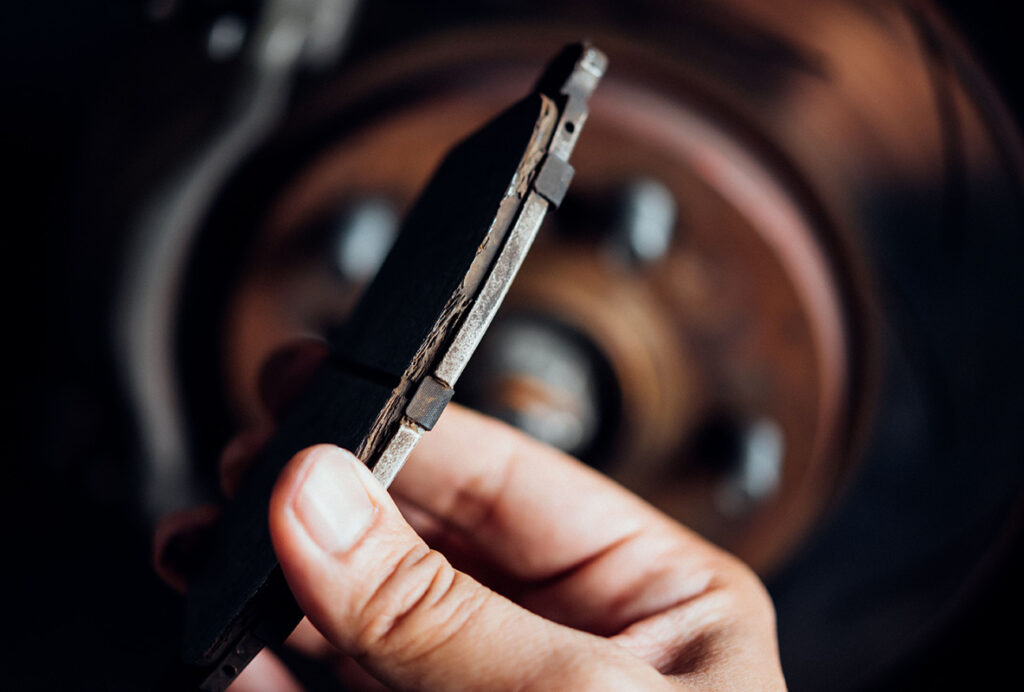
Even though external elements such as driving conditions, driving habits, and environmental factors significantly affect the durability of brake pads, their design and material composition definitely play a role.
Depending on the proportion of hard and soft abrasives, fillers, and friction modifiers used in the brake pad’s formulation, it will either be more sacrificial or stay durable at the expense of rotor wear.
Materials like rubber compounds, kevlar fibers, mica, talc, organic fibers, and silica wear out much faster than the harder stuff, such as aluminium oxide or zirconium silicate.
Noise, Judder, Vibration
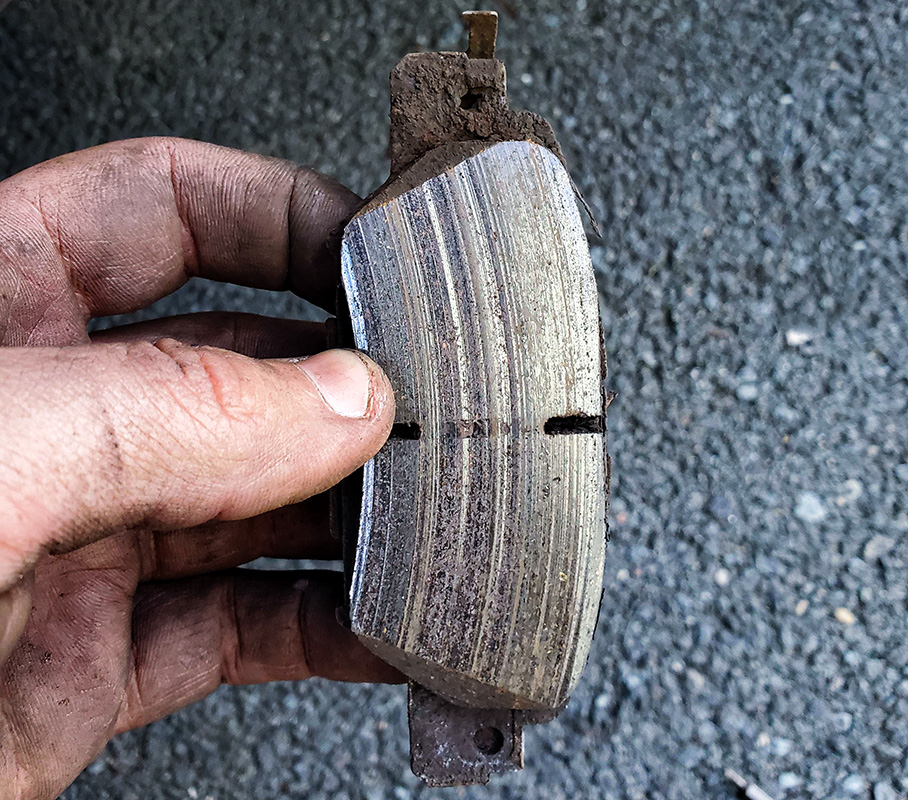
Brake pad formulations use abrasives, and with abrasives comes noise, judder, and vibration. Other factors, such as humidity, ambient temperature, and road conditions, also affect this.
Some brake pads are able to mitigate this by using mica, vermiculite, sponge iron, graphite, organic fibers, and barium sulfate. Mechanical design features such as slots and chamfers also help.
Dust Production
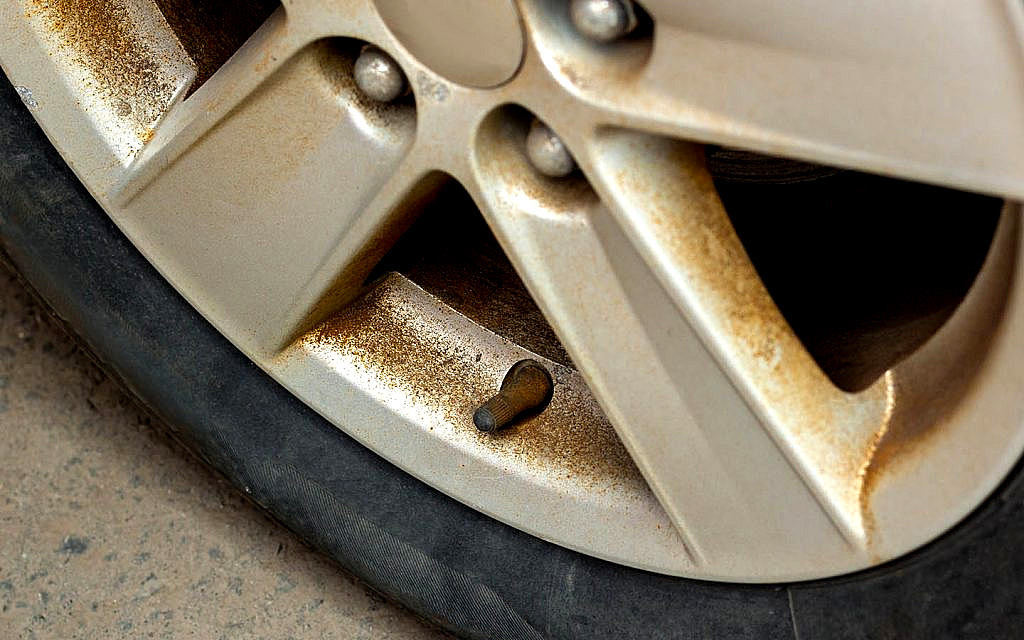
While this is a non-issue for most drivers, some raise concerns with regard to aesthetics and air quality. Politics aside, it’s an objective fact that rotors and brake pads wear in on one another and release various metals and particulates that impact air quality.
Excessive dust can also contaminate brake components, but this is a rare occurrence. If, for whatever reason, you want brake pads that produce less dust, we recommend using NAO pads that contain more calcium carbonate and graphite.
Dynamic Coefficient of Friction
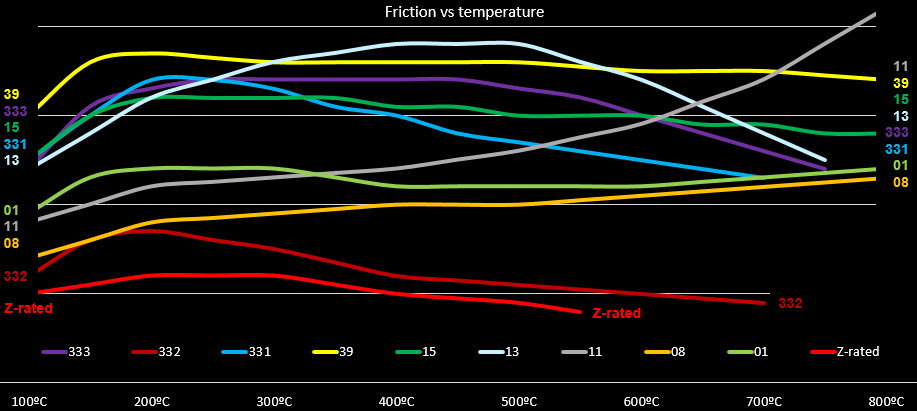
This arguably the most critical factor to understand when selecting brake pads for your car. Among all the attributes listed above, DFC is going to have the most noticeable real world effect since it directly affects brake performance and pedal feel.
In simple words, the coefficient of friction in this context is essentially a measurement of how effective the brake pad is at generating stopping force. More specifically, it is the relationship between frictional force on the pad vs the rotor.
In academic terms, the coefficient of friction ( denoted by the Greek letter mu or 𝛍) is the ratio of the resistive force of friction divided by the perpendicular force (N) pushing the pad and the rotor together.
It tells you how two surfaces interact with other when one is moving and the other is trying to keep it from moving, and it’s represented by the equation: 𝛍= Fr/N.
For example,
Force on the pad: 1000 newtons
Force on the rotor: 400 newtons
DFC(𝛍) = 0.4
For perspective, 𝛍0 denotes full lubricity with no friction at all, while 𝛍1.0 is essentially a dead stop, with no molecules moving at all. Back when brake pads were first invented, 𝛍0.20 was considered a feat of engineering. Today, those are rookie numbers.
Modern day pads range from 𝛍0.20 for your run-of-the-mill organic pads to a whopping 𝛍0.70 or higher for race applications.
Street-legal brake pads sold in the U.S. feature DOT-mandated friction codes and other data that gives you a ton of info about the brake pad’s performance. Particularly the coefficient of friction, which is further classified under SAE standard J866.
It is expressed as a 2-letter code where the first letter indicates friction at low operating temps (200F to 400F) and the second letter indicates the same at a higher temperature (300F to 650F). Higher letters in the alphabet entail better friction performance than lower letters.
Friction Coefficient Range | Code |
---|---|
Up to 0.15 | C |
Over 0.15 up to 0.25 | D |
Over 0.25 up to 0.35 | E |
Over 0.35 up to 0.45 | F |
Over 0.45 up to 0.55 | G |
Over 0.55 | H |
Unclassified | Z |
For instance, FE would indicate that the pad has an extremely high initial bite with 𝛍 between 0.35 and 0.45, and a reduced bite after warming up.
Please note that not all brake pads are marked according to these classifications. Full race pads, for example, often operate at high temperature ranges that fall outside these categories.
Some manufacturers use their own proprietary classification systems, such as color-coding the pads or employing unique numbering schemes.
Adhesive Force (Third Body Layer)
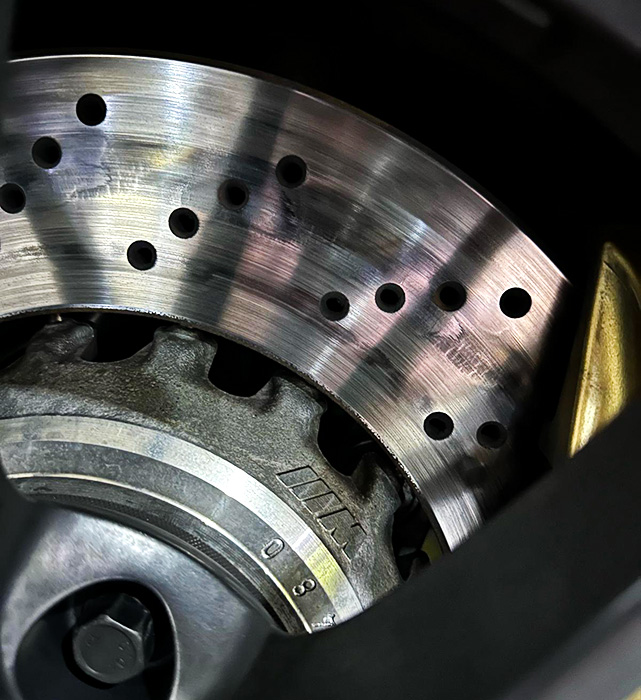
Brake pads create friction in 2 ways: abrasive and adherent.
Abrasive friction wears down the rotor since particles on both the pad friction material and the rotor wear off and fall apart. In contrast, adherent or adhesive friction is what happens when a thin layer of the pad bonds to the rotor through the process of heat and friction.
Picture molten rubber stuck to the tarmac after doing burnouts; something similar happens as the brake pad leaves some of its residue on the rotor, although it’s a very thin layer, and is referred to as a third body layer or transfer layer.
This layer gets bonded to the rotor surface because of extreme heat and there’s no way you can wash it away with water or wheel cleaners. It has to be removed on a brake lathe or by using unholy amounts of heat.
You want the third body layer on the rotor though. That’s what the pads stick to and that’s how it’s able to provide adherent friction, in addition to abrasive friction. Higher end brake pads use adherent friction because it’s less destructive to the rotor.
As mentioned earlier, the formulation of the friction material dictates the proportion of adherent or abrasive friction.
Construction: What’s Inside?
Friction materials typically comprise reinforcement material, binders, fillers (functional or inert), and friction modifiers (abrasives or solid lubricants).
These are all bound to a solid metal backing plate, which makes it easier to slot them in and out of your calipers. Here’s an overview of what’s inside brake pads.
Reinforcement Material
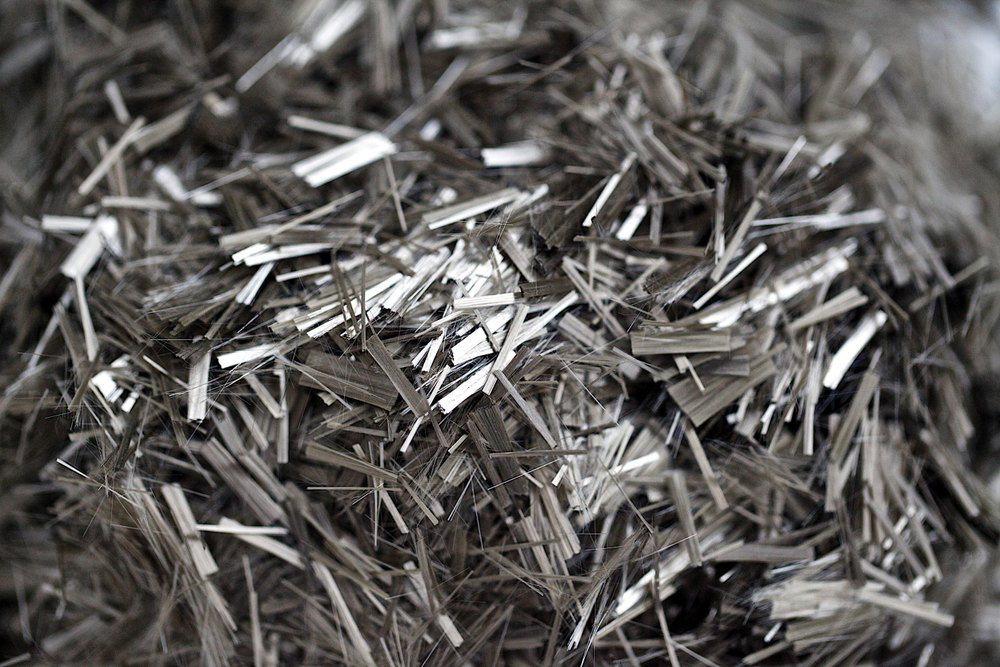
Brake pads utilize various reinforcement materials to enhance strength and durability. These materials often include fibers like aramid, steel, basalt, or ceramic particles.
Aramid fibers, known for their high tensile strength, are common in high-performance pads, while steel fibers add toughness. Ceramic particles contribute to heat resistance and stability, crucial for heavy braking.
Binder
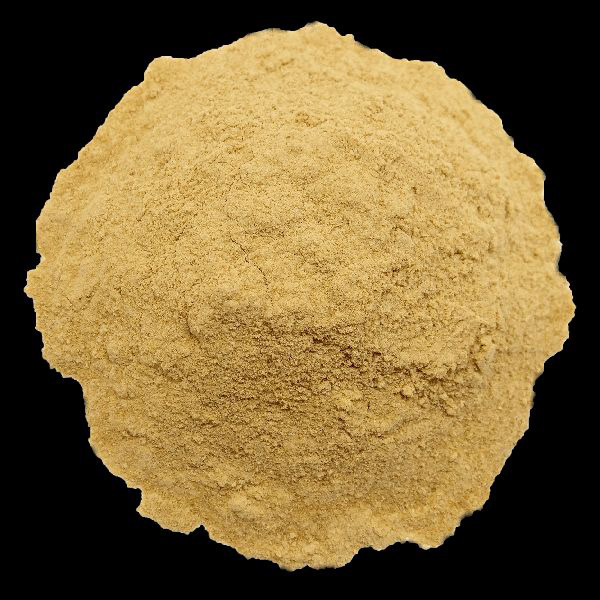
The binder in brake pads holds the friction materials together and bonds them to the backing plate. It is typically a heat-resistant resin that ensures cohesion under extreme temperatures and pressures.
The binder’s properties directly influence the pad’s performance, including its ability to withstand heat and maintain structural integrity over time.
Filler
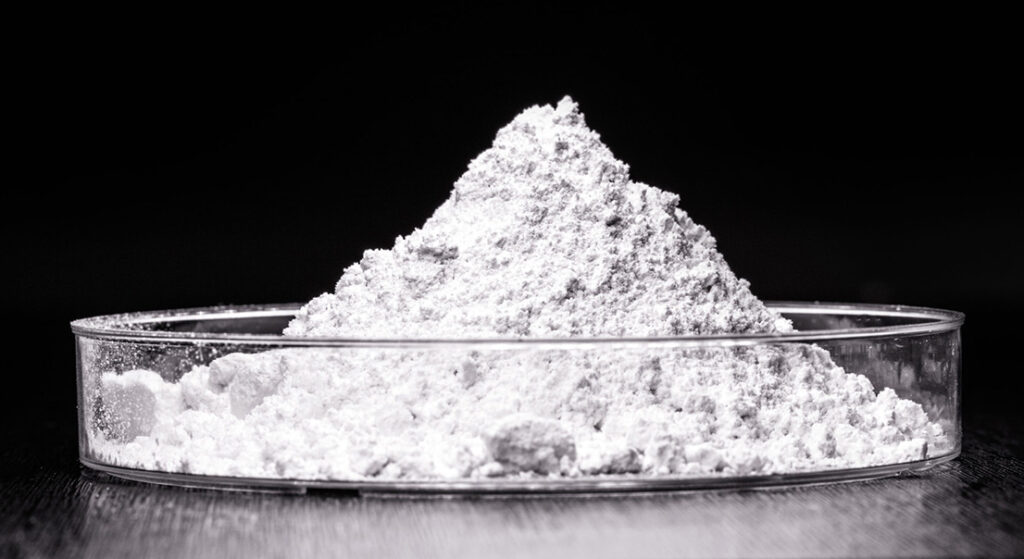
Fillers in brake pads are additives that modify the pad’s properties and performance characteristics. They play a crucial role in fine-tuning the pad’s friction, wear rate, and noise levels.
These include materials like graphite, copper, barite, calcium carbonate, mica, and vermiculite. Graphite, for instance, helps reduce noise and improve lubrication, while copper fibers enhance thermal conductivity and wear resistance.
Friction Modifier
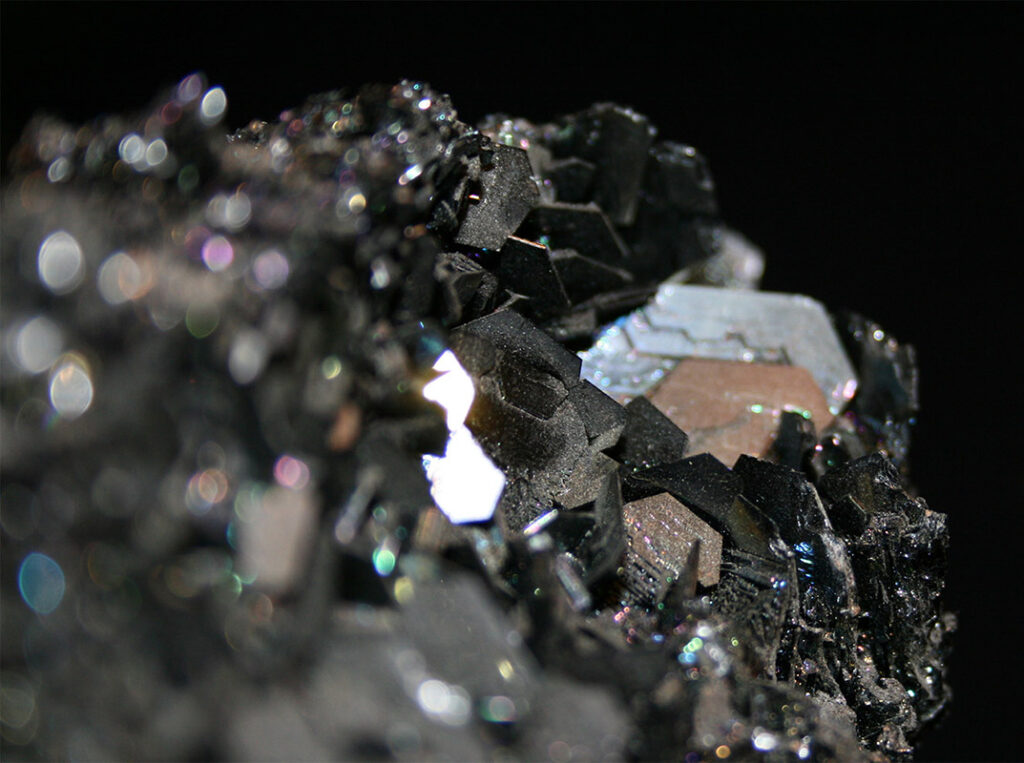
Friction modifiers are substances added to brake pads to adjust and optimize their frictional properties. They can enhance or reduce the coefficient of friction, affecting braking performance under various conditions.
Common friction modifiers broadly include abrasives, lubricants, cushioning agents. More specifically, these consist of silica, mullite, silicon carbide, metal sulfides, metal oxides, and ceramics.
Together, these modifiers help tailor the brake pad’s response to temperature changes, improve braking consistency, and mitigate issues like noise and vibration.
Types of Brake Pad Compounds
Now that you know the basics of brake pads, their construction, and the compounds used in friction materials, let’s briefly explore the different types of brake pad compounds.
These are the main differentiators you’ll come across when buying a set of pads for your car. We’ve included a spec sheet for each type to give you a quick and simple idea of what to expect from each type.
Organic
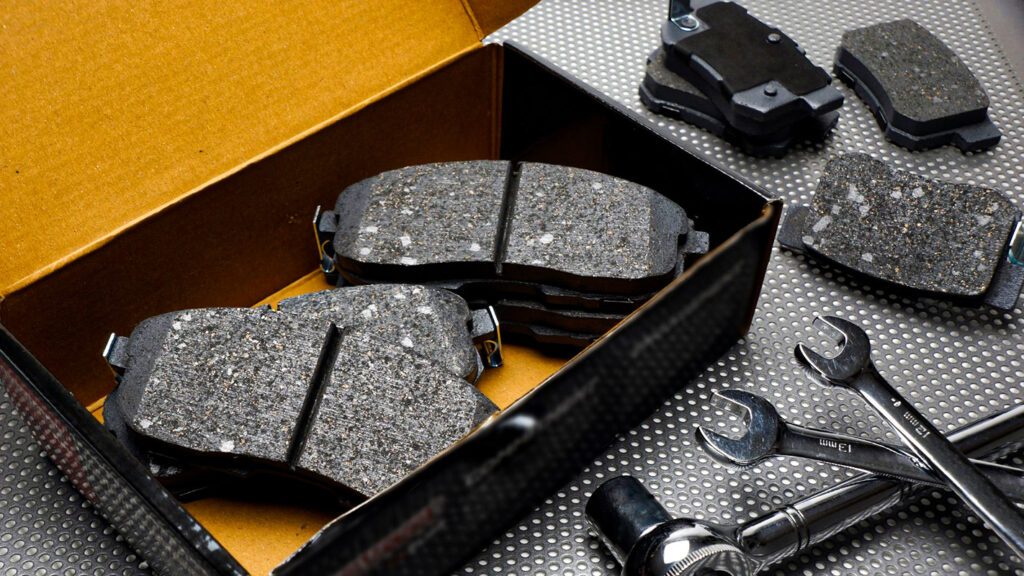
Compressibility: High
Longevity: Moderate to low
Rotor wear: Low
Noise: Low
Dust: Moderate
Application: Everyday driving, city driving
DFC range (µ): 0.3 – 0.4
Fade resistance: Low to Moderate
Operating temp range: 38°C to 204°C (100°F to 400°F)
If you want brakes that don’t break your bank, are quiet, and don’t punish your rotors, organic pads are the way to go.
These are standard equipment on most OEM cars since they’re cheap and easy to live with. However, they don’t bite as well and tend to wear out faster compared to other types mentioned below.
Their affordability and reduced environmental impact due to the absence of asbestos are key advantages, but their performance limitations make them less suitable for high performance applications.
Formulation includes metallic compounds, phenolic resin, graphite, friction dust, and a blend of fibers.
Low-Metallic
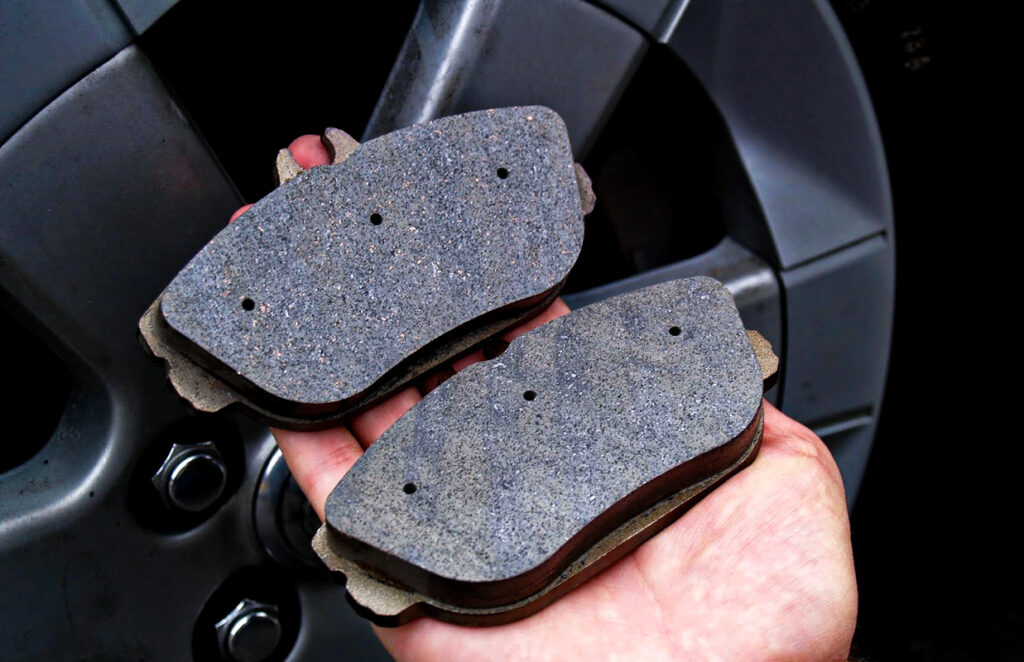
Compressibility: Moderate
Longevity: Moderate
Rotor wear: Moderate
Noise: Moderate
Dust: Moderate
Application: General-purpose driving, occasional performance use
DFC range (µ): 0.35 – 0.40
Fade resistance: Moderate to High
Operating temp range: 38°C to 427°C (100°F to 800°F)
Often considered interchangeable with low-steel, low metallic compounds, these brake pads incorporate a small percentage of metals, such as copper or steel, combined with non-asbestos-based organic materials.
This composition enhances their heat dissipation capabilities and provides better braking performance compared to fully organic pads. Unlike semi-metallic pads, low-metal and low-steel formulations often include lubricants and rubber to dampen noise and vibrations.
They also use fine, round-shaped abrasive particles to reduce wear and are commonly used on front axles where most braking load occurs.
These pads prioritize comfort over performance, making them ideal for everyday driving where reduced noise and smoother braking are required.
Semi-Metallic
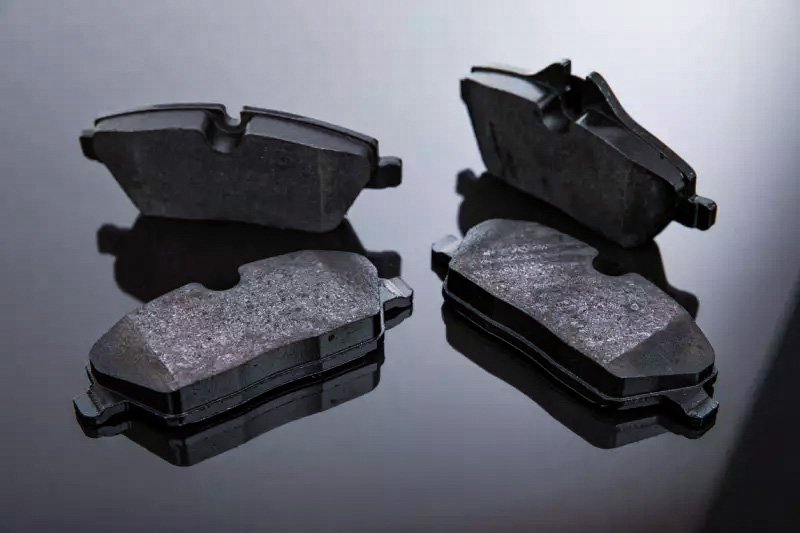
Compressibility: Moderate
Longevity: High
Rotor wear: High
Noise: High
Dust: High
Application: Performance driving, heavy-duty applications
DFC range (µ): 0.35 – 0.5
Fade resistance: High
Operating temp range: 38°C to 371°C (100°F to 700°F)
Technically still organic, semi-metallic pads were first introduced in 1939 to replace asbestos, and they’ve stuck ever since. No pun intended. They’re the most versatile option on this list, featuring the best of both, NAO and full metallic pads.
They’re made from a resin-bonded mix of metals like copper, steel, and iron powder, all held together by graphite. Performance is definitely much better than organic pads, with improved fade resistance and a higher DFC range.
However, they tend to generate more noise and dust compared to ceramic and NAO pads. It’s also worth noting that they work best when warm, so expect them to throw tantrums on cold mornings.
Sintered / Full Metallic Pads
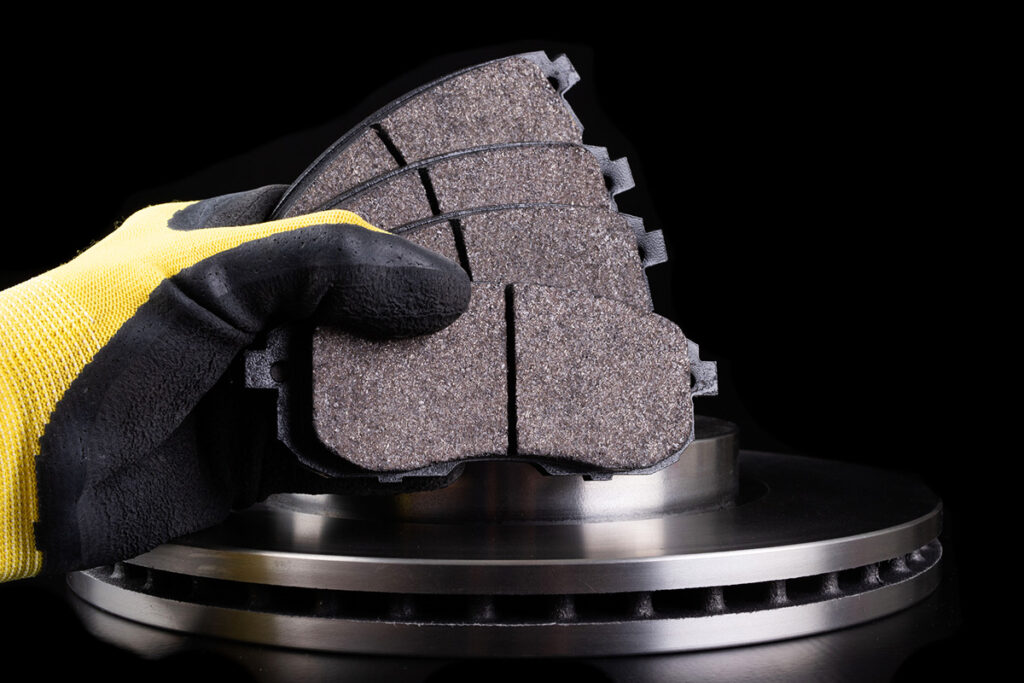
Compressibility: Low
Longevity: Very high
Rotor wear: Very high
Noise: High
Dust: High
Application: Racing, heavy-duty applications
DFC range (µ): 0.4 – 0.6
Fade resistance: Very high
Operating temp range: 38°C to 700°C (100°F to 1292°F)
These are very similar to full-metallic brake pads, with the main differentiator being their manufacturing processes. Sintered metal pads are made from metal particles that are fused together under high heat and pressure, creating a dense and durable material.
These pads excel in extreme conditions, offering outstanding heat resistance and braking performance. However, they can be noisy and produce more dust, leading to increased rotor wear.
For many, that’s not a high price to pay, considering the exceptional heat dissipation properties of these brake pads; since metal is a great conductor of heat, these pads absorb a lot of it and prevent it from percolating onto other components.
Their exceptional durability and performance make them ideal for demanding environments, though they may not be the best choice for everyday driving due to their aggressive nature.
Ceramic
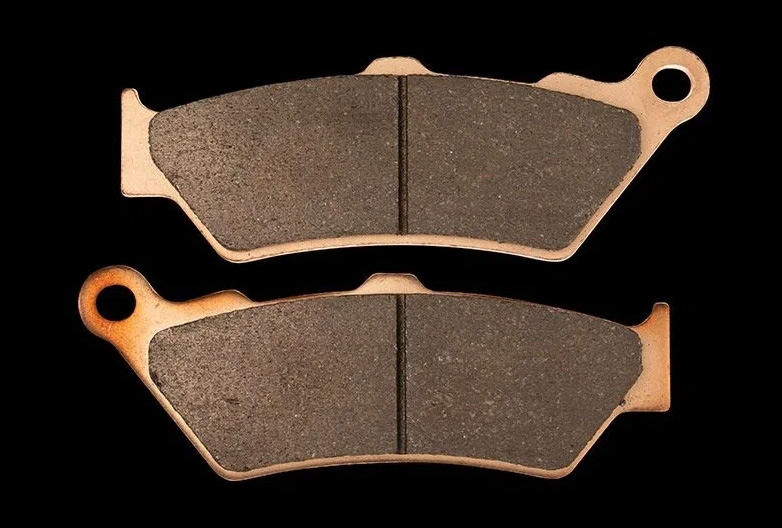
Compressibility: Low
Longevity: High
Rotor wear: Low to moderate
Noise: Low
Dust: Low
Application: Everyday driving, performance street driving
DFC range (µ): 0.35 – 0.45
Fade resistance: High
Operating temp range: 93°C to 427°C (200°F to 800°F)
Now on to the good stuff. Ceramic pads are made of a hardened-clay-like material and they contain mostly ceramic fibers and fillers. There’s very little metal content, ensuring quiet operation, low dust, and consistent performance across various temperatures.
Although, they take time to warm up and may not provide the best performance on cold mornings. They produce light dust that doesn’t stick to the wheels, which people like, and they have a much longer life than organic and semi metallic.
These are, however, considerably expensive, which explains why they’re standard on many high-end performance cars. They aren’t great at heat dissipation, which means they let a lot of the heat pass onto surrounding components, increasing wear and tear.
They don’t do too well in extremely cold weather either; if you live in a cold region, you’re better off with metallic pads.
Carbon-Ceramic
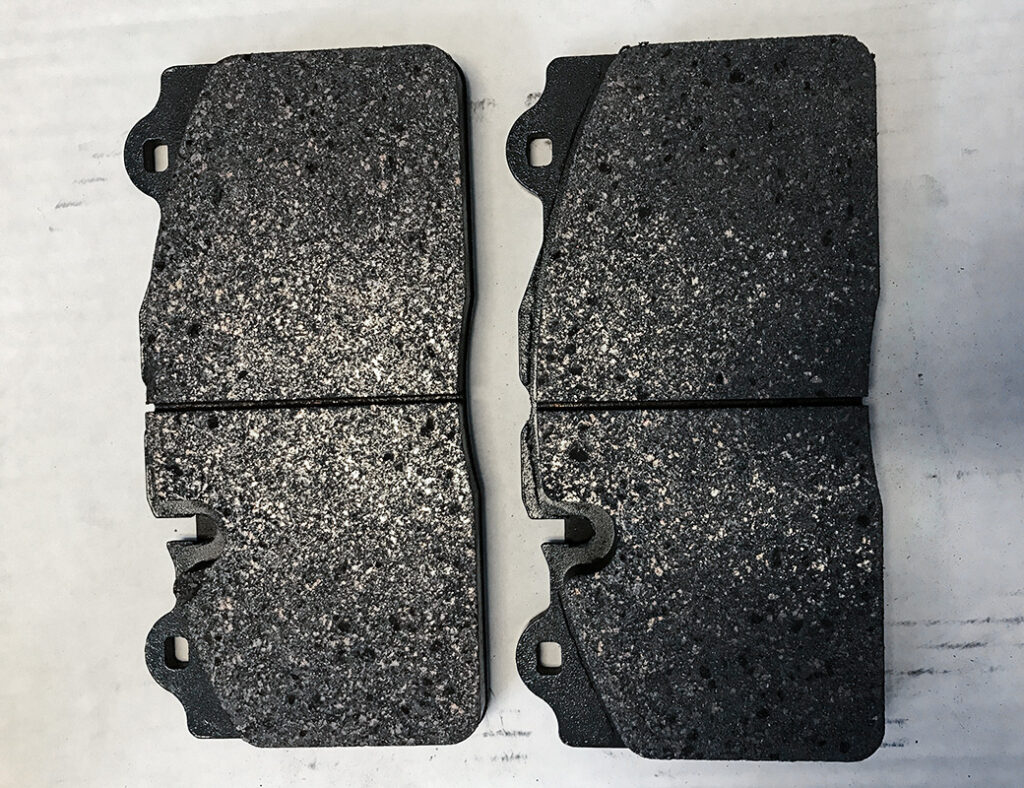
Compressibility: Very low
Longevity: Very high
Rotor wear: Low to moderate
Noise: Low
Dust: Very low
Application: High-performance racing
DFC range (µ): 0.35 – 0.45
Fade resistance: Very high
Operating temp range: 93°C to 760°C (200°F to 1400°F)
Carbon-ceramic brakes are worth a mention here, since they’re a hybrid version of ceramic brakes. They provide a lot more braking power once they’re at operating temperature, but they still have the strength and low-dust quality of regular ceramic pads.
They cause minimal rotor wear and maintain consistent performance under extreme conditions. However, they are significantly more expensive than other types of brake pads.
Reinforced Carbon-Carbon
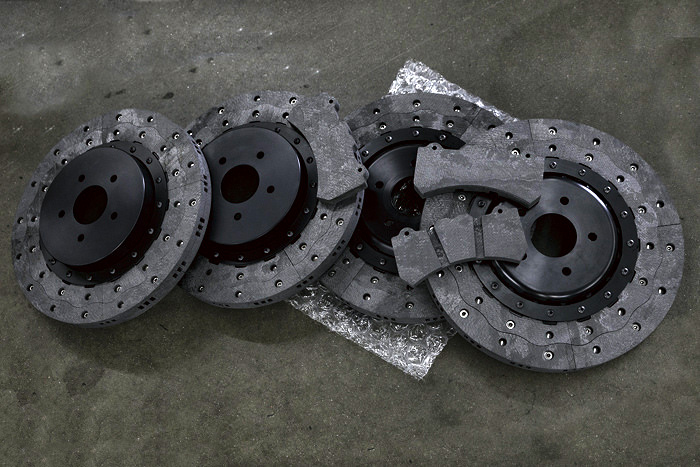
Compressibility: Very low
Longevity: Extremely high
Rotor wear: Low
Noise: Moderate to high
Dust: Very low
Application: High-performance racing, aerospace
DFC range (µ): 0.4 – 0.55
Fade resistance: Extremely high
Operating temp range: 300°C to 1500°C (572°F to 2732°F)
When it comes to the holy grail of brake pad technology (at the time of writing), carbon fiber reinforced carbon or CFRC undoubtedly takes the crown. If it’s good for high-speed trains, battle tanks, and aircraft, it’s probably good for race cars, which explains why it’s extensively used in F1.
This material was developed for the reentry vehicles (objects returning to Earth from outer space) of intercontinental ballistic missiles, and it’s most widely known as the material of choice for the nose cone and wing leading edges of the space shuttle orbiter. And to think someone put this stuff in a race car. If that’s not cool, we don’t know what is.
Concluding Thoughts
Understanding brake pad compounds is crucial for optimizing performance, handling, and safety. Each type—from organic to ceramic—offers unique benefits and trade-offs in terms of durability, noise, dust, and braking efficiency.
Choosing the right compound depends on driving habits and desired performance characteristics. What type of brake pads do you have on your car? Let us know by leaving a comment below!